LiDAR, or Light Detection and Ranging, is a technique for measuring distances using light as a pulsed laser. Combining these distances with other data captured by the Global Navigation Satellite System (GNSS) and Inertia Navigation System (INS) provide precise 3D geospatial information about objects on the planet. The high-precision surveying and mapping center is recognized as one of the most significant technological breakthroughs since the global positioning system.
Since LiDAR originated from radar, it uses lasers as its transmitting material. Lasers act similarly to radio waves except for using the laser signal as the transmission signal. In addition to high resolution, high sampling rate, concealment, strong anti-interference capability, and low-altitude detection performance, laser radar has multiple advantages.
Working principle of LIDAR
Photodiodes (PDs) within the sensor measure the reflected beam arrival time by emitting a laser beam with a defined intensity and focus. This data can determine the object’s distance in a horizontal or angular plane. This makes LiDAR similar to radar, except that it uses discrete pulses of laser light instead of a continuous beam. Coordinates of the target objects (like x, y, z, or latitude, longitude, and elevation) are computed based on:
- the time difference between the laser pulse being emitted and returned
- the angle at which the pulse was fired
- where the sensor is located about the surface of the Earth
LiDAR, unlike passive systems, emits light and detects light, making it an active approach. Due to the benefits of competitive technology, this sensor can operate in clean and harsh environments.
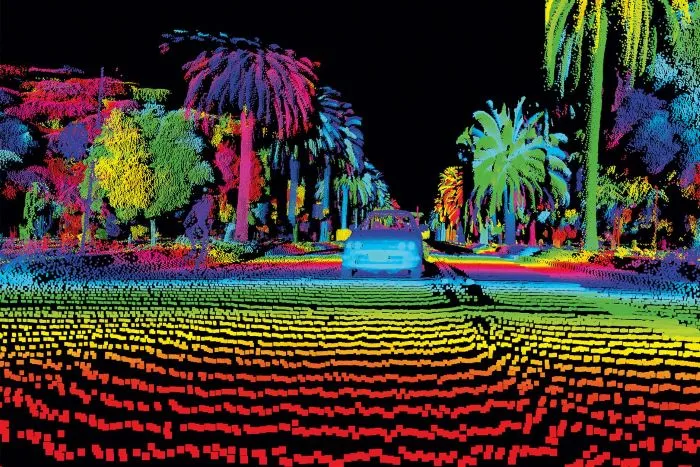
Setup
A LiDAR system’s basic configuration comprises a transmitter and a receiver. The laser produces narrow-width light pulses with spectral characteristics and durations of a few hundred nanoseconds or less.
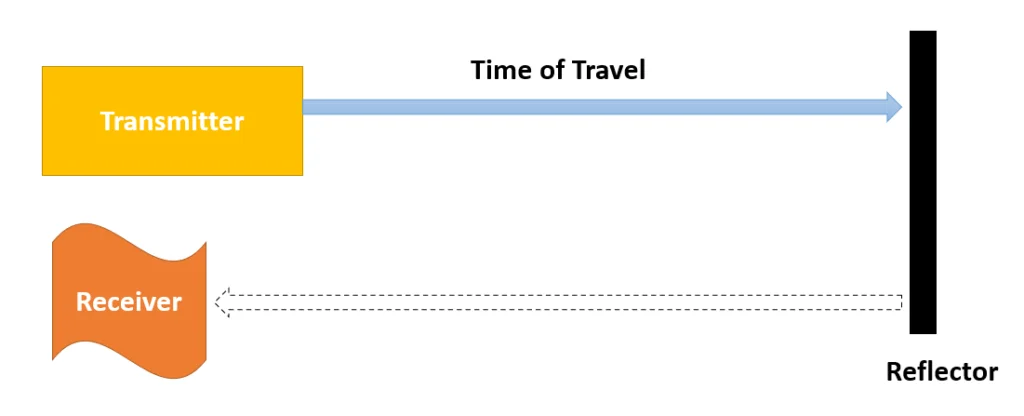
A beam expander can be incorporated into the system within the transmitter unit to lessen the divergence of the light beam before launching it into the atmosphere. The backscattered photons in the atmosphere are collected at the receiving end using a geometric optical setup. An optical analyzing system picks out particular wavelengths or polarisation states from the gathered light depending on the application. The detector receives the chosen optical wavelength radiation and converts the optical signal back into an electrical signal. The duration after the laser pulse emission determines the signal’s intensity.
The object’s distance is determined using computer-stored data and an electronic time calculation. For at least one plane, most of these devices use a single laser emitter/detector paired with a moving mirror. This mirror reflects the light emitted by the diode and received by the detector in return.
These instruments can estimate the Earth’s surface at sampling rates as high as 150 kilohertz (i.e., 150,000 pulses per second). Using this data, you can generate 3D representations of Earth’s surface and its features by constructing a densely spaced network of highly accurate elevation points known as a point cloud. Lidar wavelengths range from 250 nanometers to 11 millimeters as per the application. Excimer lasers emit ultraviolet light, whereas Nd: YAG crystals emit infrared light of 1064 nm wavelength.
LIDAR Applications
Autonomous vehicle
Autonomous vehicles or self-driving cars perceive atmospheric data in real time and produce 3D graphics using LIDAR. As part of its operation, it emits pulse-modulated light to obtain distance information and calculate angle information by using the time difference between reflected and emitted light. It offers several benefits, such as a fast response, a long detection range, and high accuracy. Its preciseness makes it the most reliable sensor solution for environmental measurements.
Due to factors such as acceleration and deceleration, changing directions, fluctuations in driving speed, and so forth, noise inevitably infiltrates the onboard LiDAR data. Presently, most onboard systems can measure the intensity of the reflected pulse, that is, how much energy is reflected from the target surface. However, when LiDAR systems, weather conditions, or other conditions differ, the laser point cloud’s intensity information will also vary. Data from the point cloud describe the surface of the object as spatial coordinates but do not specify its category.
A significant challenge arises in automatically recognizing and extracting features related to human beings and buildings in the point cloud. The cost of LiDAR makes it impractical for mass production.
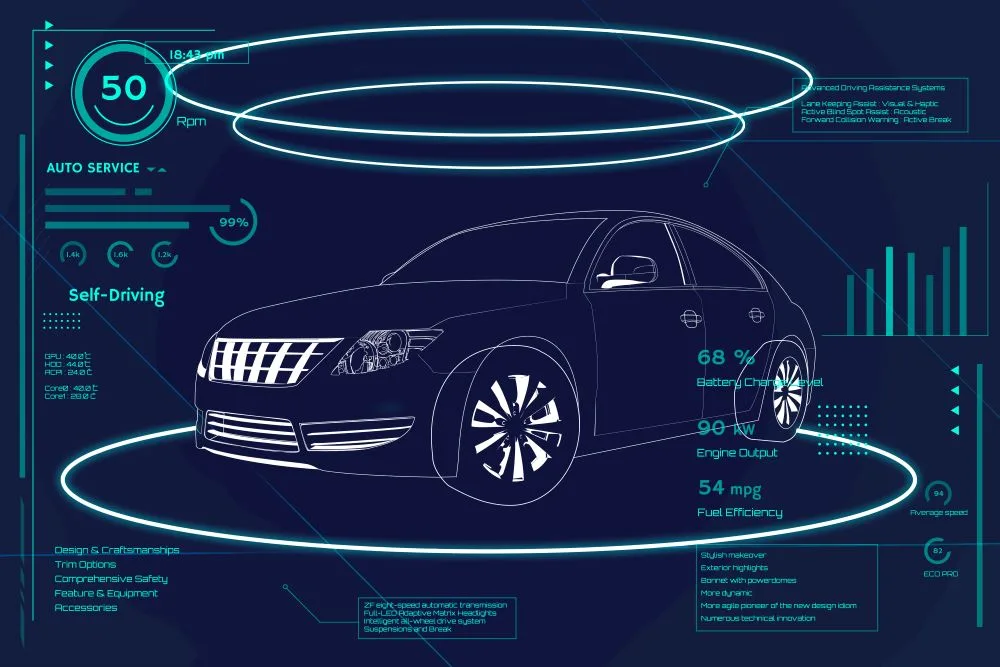
Read our article on the global impact of Electric vehicles on the automobile industry to know about this trend.
Topographic survey
Evaluating the dimensions of the forest canopy is a challenging task. LiDAR can help with measuring vegetation sizes across large sites. It also acquires data related to canopy height, upright forest design, leaf area index, recognizing species, etc. It is highly beneficial for forest management and its development. It can map forests in particular areas, know the characteristics of those forests, and assess the deterioration of forests brought on by human activity.
It is a valuable tool for gathering all the information on forest ecology, including the flora and fauna in the forests and the species that can and cannot live. Multi-spectral LiDAR captures the 3D geometry and the surface type of the ground.
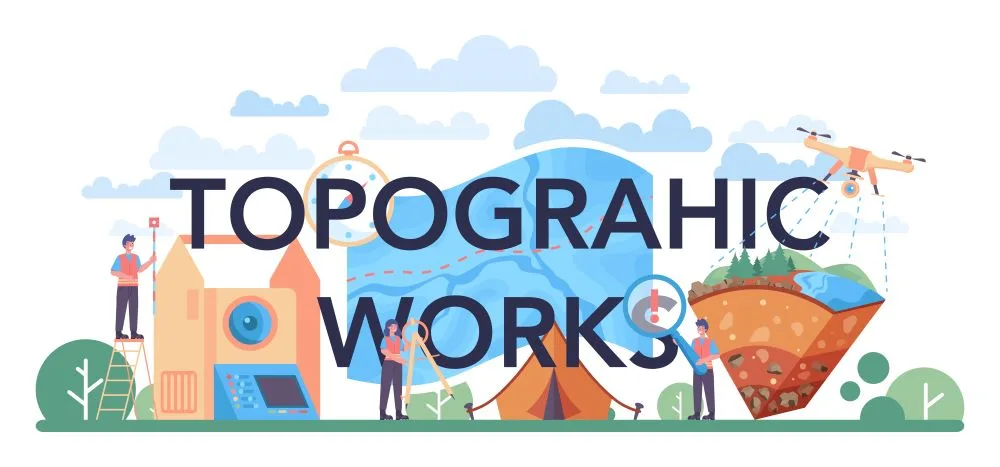
Space
A recent survey by NASA has suggested using LiDARs for complex space missions. These sensors can assess vegetation, water conditions, weather, temperature, etc., from the space perspective. A high-power narrow-line laser with stable narrow-line output is needed for DIAL LiDAR to observe water vapor and CO2 from space. These lasers are currently under development. Raman LiDAR is the only viable technique for providing fine vertical temperature profiles from space, but it requires high-power 355 nm lasers with pulse energies above 50 W.
Space-borne LiDAR systems require high-energy UV lasers, which present multiple challenges. A new development program by NASA, Surface Topography and Vegetation (STV) will speed up future space-based LiDAR development. The development of low-weight, high-electrical-to-optical efficiency lasers and large telescopes that are light and compact will benefit all space-based applications, regardless of the needs of the different types of lidars.
Ocean
Currently, there is no efficient way to observe the ocean. Passive visible sensors have gathered data about biogeochemical attributes and ocean optical characteristics but failed to find the water column’s vertical information. Ocean surface properties are only available from microwave and IR observations from space due to the strong absorption of water.
A synergistic combination of ocean-optimized space LiDAR and other ocean measurements would transform our ability to measure the oceans. From airborne platforms, lidar measurements could measure temperature, salinity, and other chemical composition of the ocean mixing layer simultaneously.
Terrain mapping
LiDAR catches 3D coordinates of the terrain easily and quickly. It can calculate the elevation of the ground with accuracy. Moreover, it shows 3D images of the elevation. Soil scientists can study patterns in spatial relationships by using detailed terrain modeling to study landform breaks and slope changes. Additionally, LiDAR surface models are used to enhance maps visually. The land is shown in 3D with data underneath all layers. When Lidar data is integrated with aerial photography, a 3D view can be created, which makes road planning, building planning, bridge planning, and river planning easier.
[…] Light detection and ranging (LIDAR): Escapes crashes and emergency braking occurrences. […]